Leading sources for squeezed light
Developing the world’s best squeezed-light sources in Hannover has been central to achieving the incredible sensitivity of the current gravitational wave detectors. GEO600 was the first detector to routinely employ non-classical light, and QuantumFrontiers researchers hold the record for the highest degree of fixed-quadrature squeezing (6 dB) in a full-scale gravitational wave detector to date [1]. They built and installed the fixed-quadrature squeezed-light source for Advanced Virgo [2, 3], leading to increased astrophysical reach [4], and provided crucial input to the high-power laser system for aLIGO [5, 6], leading to the detectors’ sensitivity that enabled the first direct detection of gravitational waves and allowed these detectors to operate in unison as part of the global detector network.
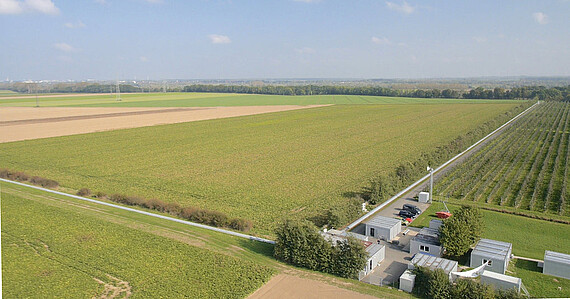
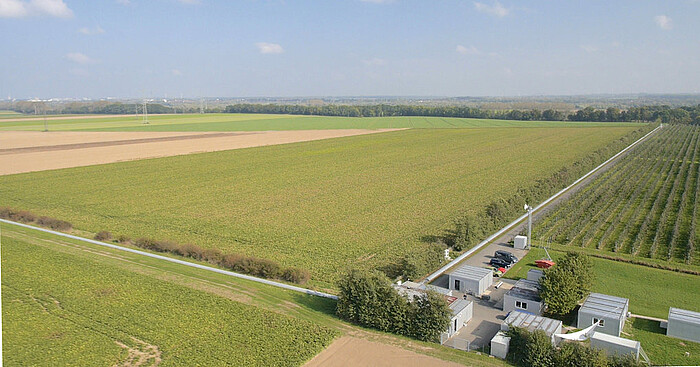
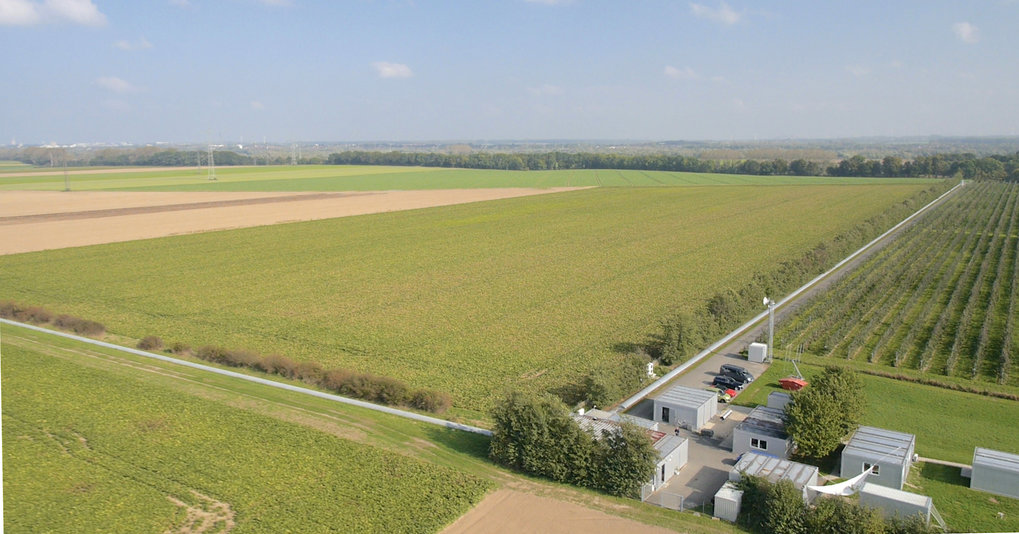
To enable future gravitational wave detectors with enhanced sensitivity, poised for making groundbreaking astrophysical and fundamental physics discoveries, QuantumFrontiers researchers have produced frequency-dependent squeezed light without external filter cavities [7], have achieved a noise reduction of over 13 dB at the laser wavelength of 1550 nm [8] and have observed squeezing in higher-order transversal modes. [9]
Research on the next generation of gravitational wave detectors
Significant advances have also been made in the coherent reduction of quantum radiation pressure noise, with the first integration of optical and optomechanical subsystems [10] on the horizon. The AEI 10 m Prototype, a large-scale facility featuring a Fabry-Pérot Michelson interferometer operating at the standard quantum limit (SQL), will provide the infrastructure to test these novel quantum techniques. For the highly successful LISA Pathfinder mission [11], QuantumFrontiers researchers developed optical sensing for test mass displacement readout [12], studied interferometric readout in labs, and monitored daily operations. They addressed optical noise sources [13] and tilt-to-length coupling noise [14]. Their contributions were crucial, demonstrating critical hardware and providing essential scientific understanding.
This article is part of a series on QuantumFrontiers success stories
These achievements have set the LISA mission on track for a mid-2030s launch, with QuantumFrontiers scientists being responsible for the interferometric detection system, phasemeter development, and noise performance studies. “Beyond LISA” efforts include miniaturising optical sensors [15], developing a torsion balance as a force testbed [16], and mitigating optical noise sources [17].
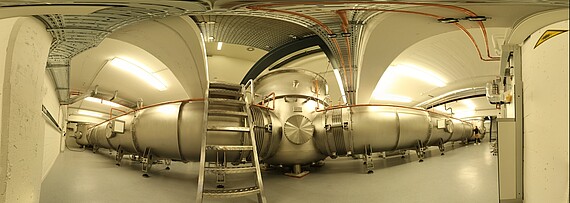
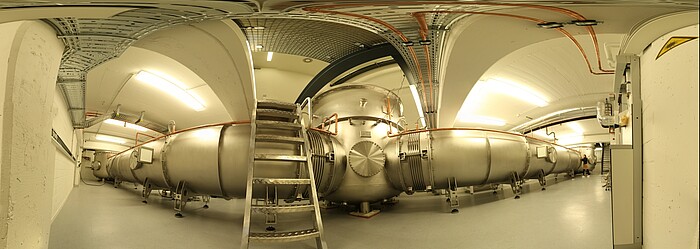
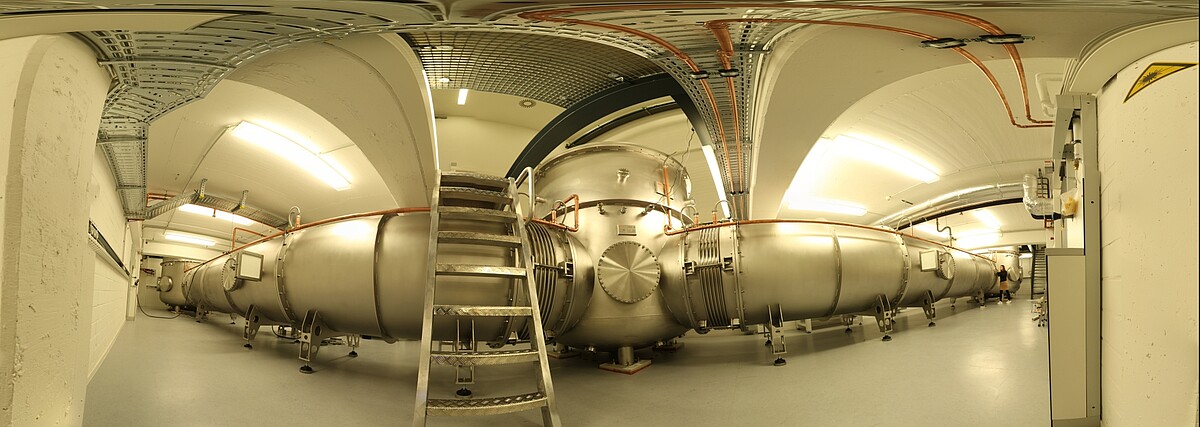
Finally, principal investigators from QuantumFrontiers play a key role in infrasound GWD using matter-wave interferometry. In the terrestrial VLBAI collaboration, various detector concepts were explored [18] with partners in the USA (MAGIS) and China (ZAIGA). 10-meter-scale prototypes are operationally ready, including the VLBAI facility in Hannover. Under European Laboratory for Gravitation and Atom-interferometric Research (ELGAR), QuantumFrontiers researchers are preparing studies for a pan-European infrastructure for a 3D infrasound GWD antenna, including squeezed atomic sources.
Publications
-
Bibliography
[1] J. Lough, E. Schreiber, et int, K. Danzmann. First Demonstration of 6 dB Quantum Noise Reduction in a Kilometer Scale Gravitational Wave Observatory.
Physical Review Letters 126. doi:10.1103/physrevlett.126. 041102 (2021).[2] F. Acernese, M. Agathos, et int, K. Danzmann. Increasing the Astrophysical Reach of the Advanced Virgo Detector via the Application of Squeezed Vacuum States of Light.
Physical Review Letters 123. doi:10.1103/physrevlett.123.231108 (2019).[3] M. Mehmet, H. Vahlbruch. The Squeezed Light Source for the Advanced Virgo Detector in the Observation Run O3.
Galaxies 8, 79. doi:10.3390/galaxies8040079 (2020).[4] F. Acernese, M. Agathos, et int, K. Danzmann. Frequency-Dependent Squeezed Vacuum Source for the Advanced Virgo Gravitational-Wave Detector.
Phys. Rev. Lett. 131, 041403. doi:10.1103/PhysRevLett.131.041403 (2023).[5] F. Wellmann, N. Bode, et int, D. Kracht. Low noise 400 W coherently combined single frequency laser beam for next generation gravitational wave detectors.
Optics Express 29, 10140. doi:10.1364/oe.420350 (2021).[6] F. Wellmann, M. Steinke, et int, D. Kracht. Performance study of a high-power single-frequency fiber amplifier architecture for gravitational wave detectors.
Appl. Opt. 59, 7945. doi:10.1364/ao.401048 (2020).[7] J. Junker, D. Wilken, et int, M. Heurs. Frequency-Dependent Squeezing from a Detuned Squeezer.
Physical Review Letters 129. doi:10.1103/physrevlett.129.033602 (2022).[8] F. Meylahn, B. Willke, H. Vahlbruch. Squeezed States of Light for Future Gravitational Wave Detectors at a Wavelength of 1550 nm.
Phys. Rev. Lett. 129, 121103. doi:10.1103/PhysRevLett.129.121103 (2022).[9] J. Heinze, B. Willke, H. Vahlbruch. Observation of Squeezed States of Light in Higher-Order Hermite-Gaussian Modes with a Quantum Noise Reduction of up to 10 dB.
Phys. Rev. Lett. 128, 083606. doi:10.1103/PhysRevLett.128.083606 (2022).
[10] J. Schweer, D. Steinmeyer, K. Hammerer, M. Heurs. All-optical coherent quantum-noise cancellation in cas-caded optomechanical systems.
Physical Review A 106. doi:10.1103/physreva.106.033520 (2022).
[11] M. Armano, H. Audley, et int, P. Zweifel. Sub-Femto-g Free Fall for Space-Based Gravitational Wave Observatories: LISA Pathfinder Results.
Physical Review Letters 116. doi:10.1103/physrevlett.116.231101 (2016).
[12] M. Armano, H. Audley, et int, P. Zweifel. Sensor Noise in LISA Pathfinder: In-Flight Performance of the Optical Test Mass Readout.
Physical Review Letters 126. doi:10.1103/physrevlett.126.131103 (2021).
[13] M. Armano, H. Audley, et int, P. Zweifel. Sensor noise in LISA Pathfinder: An extensive in-flight review of the angular and longitudinal interferometric measurement system.
Physical Review D 106. doi:10.1103/physrevd.106.082001 (2022).[14] M. Armano, H. Audley, et int, P. Zweifel. Tilt-to-length coupling in LISA Pathfinder: A data analysis.
Physical Review D 108. doi:10.1103/physrevd.108.102003 (2023).[15] K.-S. Isleif, G. Heinzel, M. Mehmet, O. Gerberding. Compact Multifringe Interferometry with Subpicometer Precision.
Physical Review Applied 12. doi:10.1103/physrevapplied.12.034025 (2019).[16] G. Bergmann, C. Cordes, et int, M. Mehmet. A torsion balance as a weak-force testbed for novel optical inertial sensors.
Classical and Quantum Gravity 41, 075005. doi:10.1088/1361-6382/ad29e8 (2024).[17] M.-S. Hartig, S. Schuster, G. Heinzel, G. Wanner. Non-geometric tilt-to-length coupling in precision interferometry: mechanisms and analytical descriptions.
J. of Optics 25. doi:10.1088/2040-8986/acc3ac (2023).[18] S. Abend, B. Allard, et int, E. Zupanič. Terrestrial Very-Long-Baseline Atom Interferometry: Workshop Summary.
arXiv: 2310.08183 [hep-ex] (2023).